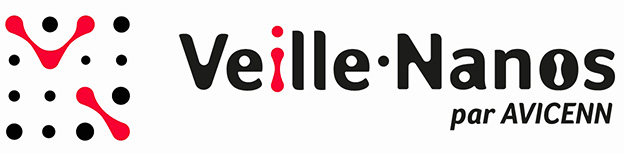
Entry points and fate of nanomaterials in the human body
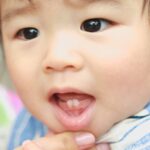
Entry points and fate of nanomaterials in the human body
By the AVICENN team – Last modification October 2024
Because of their small size, nanomaterials can enter the human body through different channels:
– the air we breathe,
– the food we ingest,
– products that we apply on skin.
The small size of nanomaterials also explains their propensity to diffuse in the body through the nervous, blood and lymphatic systems.
The three main routes of exposure to nanomaterials:
There are three main routes of potential exposure to nanomaterials:
Inhalation
Inhalation is the main route of penetration of nanomaterials in the human body1See for example:
– Human study reveals nanoparticles cross from lungs into blood – Gold nanoparticles accumulate in arterial plaques, Chemical Watch, May 2017 (see Inhaled Nanoparticles Accumulate at Sites of Vascular Disease, Miller MR et al, ACS Nano, 11(5): 4542-4552, April 2017)
– Uranium nanoparticles cross the pulmonary barrier, Aktis (IRSN), October-December 2013
– Les nanomatériaux, INRS, ED6050, September 2012.. This is especially true for workers involved in the manufacture or handling of nanomaterials in powder form.
The nanomaterials likely to be inhaled by the general public are those contained in powder form or in sprays of household products, sun creams or spray paints for example2See Potential exposure of adults and children to particles from resuspended nano-enabled consumer sprays, He R at al., Science of The Total Environment, 924, May 2024.
Once inhaled, nanomaterials can be released or remain in the respiratory tract (in the nasal cavity, bronchial tubes and pulmonary alveoli) or be carried into the gastrointestinal tract after swallowing.
Nanomaterials with diameters between 10 and 100 nm penetrate more deeply in the pulmonary alveoli than the micrometric particles and then pass, in part, into the blood, which then carries them to the other organs; on the other hand, smaller nanomaterials tend to remain in the upper airways3See Les nanomatériaux, INRS, ED6050, September 2012 and can:
- be expelled (sneezing, nose blowing)
- be swallowed and pass into the digestive system and then into the whole body
- enter the nerve endings lining the nasal cavity, then travel up the neural pathways and enter the brain via the olfactory bulb.
Ingestion
Present in our food, in medicines, in toothpastes, lipsticks or lip balms, nanomaterials can be found in the gastrointestinal system.
The smaller the nanomaterials, the more they seem to be absorbed by the digestive system and then dispersed in the body to the liver, spleen, intestines, endocrine glands and brain.
- In what proportion? This depends on the nanomaterials and the conditions under which they evolve in the body. Thus, scientists estimate that:
- 90 to 95% of the ingested TiO₂ should be released in the feces4Cf. Oral exposure to titanium dioxide (TiO2) nanoparticles: from crossing the oral and intestinal epithelium to fate and effects in the body, Bettini S and Houdeau E, Biology Today, September 2014 but even if the remaining fraction is small, one should not neglect the accumulation effect, given the high exposure throughout life, which can lead to cumulative doses potentially harmful to the organism; recent publications have thus confirmed the presence of nanoparticles of titanium dioxide (TiO2) in the liver and spleen of 15 humans (not only on laboratory rats). In half of the cases, the levels were above the level considered safe for the liver.
- Zinc oxide (ZnO) appear to be more strongly absorbed by the body because it is better dissolved in the gastric environment.
- Nanoparticles tend to agglomerate in aqueous or acidic media and regain individuality in basic media. For example, silica or silver nanoparticles isolated in saliva agglomerate after acidification in the stomach but are found again in particulate form in the intestine at neutral pH5See in particular:
For the money:
– Yves-Jacques Schneider in Nanoparticles dans nos assiettes: le Grand Secret, RTS, 3 May 2016 (intervention 19′)
– Walczak AP et al, Behaviour of silver nanoparticles and silver ions in an in vitro human gastrointestinal digestion model, Nanotoxicology, 7(7), 2012
For silica:
– Eric Houdeau and Marie-Hélène Ropers (INRAE), Nanomaterials in food. What functions and applications? What are the risks?, NanoResp, October 2015
– Peters R & Bouwmeester H, Fate of nano-sized silica during in vitro digestion, RIKILT, 2013.
Skin contact
Nanomaterials present in cosmetics or clothing, for example, can come into contact with our skin.
Penetration of nanomaterials through the skin – sometimes specifically intended for therapeutic applications6See for example Nanotherapeutic approaches for transdermal drug delivery systems and their biomedical applications, Khan SU et al., European Polymer Journal, 207, 2024 or niche cosmetics7See for example “Our cosmetics are worth their weight in gold,” Avantages Magazine, December 7, 2018: “By passing through the skin barrier, gold would fight against oxidative stress due to pollution and UV, thus limiting wrinkles. It would also be able to fight against spots and boost the skin’s immune system.” – is, in most other cases, rather dreaded. It is supposed to be somewhat limited but it should not be underestimated8Cf. for example:
– Ex vivo skin diffusion and decontamination studies of titanium dioxide nanoparticles, Tarantini A et al., Toxicology in Vitro, 101, 105918, 2024
– A critical review investigating the use of nanoparticles in cosmetic skin products, Piluk T et al., Environ. Sci.: Nano, 11, 3674-3692, 2024
– SCCS Guidance on the safety assessment of nanomaterials in cosmetics, Scientific Committee on Consumer Safety (SCCS), 2nd revision, June 2023
– Toxicity assessment of nanoparticles in contact with the skin, Zaiter T et al., Journal of Nanoparticle Research, 24 (149), 2022
– A critical review of the factors determining dermal absorption of nanomaterials and available tools for the assessment of dermal absorption, Triskelion & RIVM for EUON, 2020
– Toxicity evaluation of TiO2 nanoparticles on the 3D skin model: a systematic review, Sanches PL et al., Front Bioeng Biotechnol, 8, 2020
– NanoTiO2 Sunscreen Does Not Prevent Systemic Oxidative Stress Caused by UV Radiation and a Minor Amount of NanoTiO2 is Absorbed in Humans, Pelclova D et al., Nanomaterials, 9(6), 888, 2019
– NanoTiO2 Sunscreen Does Not Prevent Systemic Oxidative Stress Caused by UV Radiation and a Minor Amount of NanoTiO2 is Absorbed in Humans, Pelclova D et al, Nanomaterials, 9(6), 888, 2019
– Support for the Safe Use of Zinc Oxide Nanoparticle Sunscreens: Lack of Skin Penetration or Cellular Toxicity after Repeated Application in Volunteers, Mohammed YH, Journal of Investigative Dermatology, 139(2): 308-315, February 2019
– Sunscreen, nanoparticles and frontal alopecia, Synchrotron sun, press release, February 2018 (see also academic article in English: Postmenopausal fibrosing frontal alopecia: a lichenoid reaction to titanium dioxide nanoparticles in hair follicles?, Gary C et al., Annals of Dermatology and Venereology, 144 (12), S206, 2017)
– Skin exposure to nanoparticles and possible sensitization risk, Larese Filon in Allergy and Immunotoxicology in Occupational Health, Otsuki, T, Petrarca, C, Di Gioacchino, M (eds) 143-152, 2017
– Titanium dioxide and silver nanoparticles- Dermal exposure, Proust N, Techniques de l’Ingénieur, January 2017
– Bioengineered sunscreen blocks skin penetration and toxicity, NIBIB, December 2015
– Is the skin really impervious to nanoparticles, Vinches L and Halle S, Bulletin de veille scientifique, No. 27, ANSES, September 2015
– A review of critical factors for assessing the dermal absorption of metal oxide nanoparticles from sunscreens applied to humans and a research strategy to address current deficiencies, Gulson, B, Arch. Toxicol. 89, 1909–1930, 2015
– Nanoparticles skin absorption: New aspects for a safety profile evaluation, Larese Filon F et al. Regulatory Toxicology and Pharmacology, 2015: according to this literature review:
. nanoparticles smaller than 4 nm in diameter can penetrate and impregnate intact skin,
. nanoparticles with a diameter between 4 and 20 nm can potentially penetrate intact and injured skin,
. nanoparticles with a diameter between 21 and 45 nm can only penetrate and impregnate injured skin,
. nanoparticles with a diameter greater than 45 nm cannot penetrate or impregnate the skin.
– Other aspects play an important role, especially for metallic nanoparticles, namely their dissolution in physiological media, which can cause local and systemic effects, their sensitizing or toxic potential and the tendency to create aggregates.
– Interactions of Skin with Gold Nanoparticles of Different Surface Charge, Shape, and Functionality, Fernandes R et al, Small, October 2014
– Dermal exposure potential from textiles that contain silver nanoparticles, International Journal of Occupational and Environmental Health, 20(3), July 2013
– Dermal Absorption of Nanomaterials, Danish Environmental Protection Agency, 2013
– Occupational exposure to nanoparticles and skin protection, Archives of Occupational and Environmental Diseases, 74(5), 488-498, November 2013
– Reigniting the controversy over the ability of nanoparticles to cross the skin barrier, veillenanos.co.uk, 3 October 2012
– Titanium dioxide and zinc oxide nanoparticles in cosmetic products: State of knowledge on skin penetration, genotoxicity and carcinogenesis – Information point AFSSAPS, June 14, 2011 (see pp. 28-29 of the state of knowledge report for a presentation of the Gulson study): the French Agency for the Sanitary Safety of Health Products (Afssaps) noted that scientific studies did not show significant cutaneous penetration of titanium dioxide (TiO2) nanoparticles for healthy skin, but did not allow for any conclusion in either direction for damaged skin. Afssaps has therefore recommended not to apply creams containing nano TiO2 on injured skin (for example, sunburn) because of the potential risks to human health; it has also advised against using cosmetics containing nanoparticles in spray form on the face or in closed premises. Robust studies on the subject are still all too rare. The available results are often contradictory and not very usable due to insufficient physicochemical characterization of nanomaterials and/or the diversity of experimental setups9A synthesis was published in May 2020: Are nanomaterials getting under your skin? RIVM & RPA consortium of Triskelion, ECHA, EUON, May 2020: experts from the Netherlands commissioned by the European Chemicals Agency (ECHA) to analyze research on dermal absorption of nanomaterials highlight the lack of comparable and quality data and recommend well-organized and structured research programs in line with the OECD testing guidelines.
A study, also published in May 2020, reports interesting results: Penetration of Zinc into Human Skin after Topical Application of Nano Zinc Oxide Used in Commercial Sunscreen Formulations, Holmes AM et a., ACS Appl. Bio Mater., 2020.
Overall, the literature nevertheless suggests that the penetration rate of nanoparticles may be higher than for larger particles, which are normally blocked by the upper layers of the epidermis.
Many uncertainties remain and work is in progress, particularly in France10Research work is underway in 2021 at the CEA in Grenoble in particular, on the development of an experimental model for the study of skin decontamination after exposure to metal nanoparticles (NaPeauLi), funded under the 2019 Anses APR-EST., to clarify these aspects.
Their passage through the skin could be facilitated by sebum, sweat, repeated bending of the skin, as well as by skin lesions (in case of eczema or pimples, burns due to a sunburn, micro-cuts resulting from shaving, etc.). In this case, it cannot be excluded that nanoparticles can be distributed in the body and reach internal tissues or even other organs – in a supposedly low proportion that could be significant in the case of frequent and chronic applications.
The fate of nanoparticles in hair follicles raises questions because the presence of stem cells, which can migrate, could make it possible to transport nanoparticles inside the body via this channel. Dermatologists from the Bichat and Rothschild Hospitals observed at the Sun Synchrotron the presence of titanium dioxide (TiO2) nanoparticles along the hair follicles of a patient suffering from fibrosing frontal alopecia (hair loss at the top of the forehead) who had used daily, for 15 years, sunscreens containing TiO211Cf. Sunscreen, nanoparticles, and frontal alopecia, Synchrotron sun, February 2018.
Other routes of exposure :
Actually, there are many more routes of exposure to nanomaterials:
Urogenital tract
Nanomaterials such as nanosilver are used in the composition of underwear, antibacterial and spermicidal vaginal gels12See our inventories of marketed products containing nanomaterials, or for example: Testing for HIV with the naked eye or countering it with a cream? January 2014 and Use of silver nanoparticles increased inhibition of cell-associated HIV-1 infection by neutralizing antibodies developed against HIV-1 envelope proteins, Journal of Nanobiotechnology, 9:38, 2011. Do they then cross the physiological barriers? Avicenn has not identified any studies on this topic.
Skin breakdown
Several teams of researchers have warned about the risks linked to the transfer of nanoparticles contained in tattoo inks and/or needles to the blood, lymphatic vessels and nodes (leading to their chronic swelling) and various organs, which can cause hypersensitivity or allergy reactions13See in particular:
– Tattoo : nanoparticles, I have you in my skin, Science & Vie, 20 October 2021
– Tattoo inks and permanent make-up, ECHA, 2020 ?
– After the ink… Tattoos: needles could cause allergies, Allô Docteurs, 27 August 2019 and Metal particles abraded from tattooing needles travel inside the body, Synchrotron de Grenoble, 26 August 2019
– Scientists find that nanoparticles from tattoos travel inside the body, ESRF, September 12, 2017 (Synchrotron-based ν-XRF mapping and μ-FTIR microscopy enable to look into the fate and effects of tattoo pigments in human skin, Schreiver I et al, Scientific reports, 2017); professionals have responded by downplaying the risks, while still denouncing “the vagueness maintained by the industrialists supplying the pigments (CI = Color index) to the ink manufacturers, under cover of commercial secrecy. Our national distributors, respectful of the requirements imposed by the law, do not have themselves details on the CI of the labels. It is indeed today the only zone of shade on our tattoo inks. The real evolution expected from now on by the tattooers and the tattooed aims at a bigger transparency of the pigments suppliers… For a better knowledge of tattoo products”. Nanoparticles : Don’t panic, Tattoo Magazine, n°119, November-December 2017
– Size and metal composition characterization of nano- and microparticles in tattoo inks by a combination of analytical techniques, Bocca B et al, J. Anal. At. Spectrom.,32, 616-628, January 2017
– University of Bradford (UK) press release and Friends of the Earth Australia article: Nanoparticles in tattoos could cause cancer, 4 November 2013.
Parenteral (intravenous, vaccines, etc.)
This route is used in medicine: it is the intravenous, subcutaneous, intradermal or intramuscular routes by which nanomaterials can be introduced into the human body.
Implantable medical devices with nanocoatings are also tested or developed (pace-makers, prostheses).
Nanoparticles are also present in vaccines:
- some through non-voluntary contamination14See in particular:
–New Quality-Control Investigations on Vaccines: Microand Nanocontamination, Gatti AM and Montanari S, International Journal of Vaccines and Vaccination, 4(1), 2017
-Comparative study of particle and element testing in vaccines and other injectable health products, ANSM, May 2016, but in such minute numbers that it could, according to the European Medicines Agency, be found “everywhere in the environment” and “should not be considered a health risk15“Are vaccines “contaminated by toxic nanoparticles”?, Le Monde, July 19, 2017. - others for prophylactic (preventive) or therapeutic purposes16See for example:
– Vaccine leads under study against covid-19, some examples of which are compiled on our Nano and covid-19 page
– Nanotechnologies to produce vaccines faster, Le Soir, November 8, 2021
– Vaxinano, a biotechnology company established in 2016, specializes in the preclinical and pharmaceutical development of prophylactic and therapeutic vaccines for infectious diseases for the human and animal health markets.
– Glycovax Pharma files patent application for new semi-synthetic breast cancer vaccine, Glycovax Pharma, April 4, 2018
– Vaxinano develops first toxoplasmosis vaccine, Les Echos, Sept. 5, 2017
– A method for vaccines to no longer need cold storage, Sciences & Avenir, December 2016
– Applications of nanotechnology to medicine, LEEM, February 13, 2014
– Vaccines based on nanoparticles, Les Echos, October 3, 2007, mainly at the research and development stage, and some are already being marketed..
Oral mucosa (toothpastes, oro-dispersible medicines, chewing gums, etc.)
The oral mucous membranes are permeable; this is why granules and other so-called “oro-dispersible” drugs must be placed under the tongue (this is called a “sublingual” mode of administration) to “melt” there and be rapidly absorbed by the body. A part of the nanoparticles contained in toothpastes, medicines or chewing gums is “absorbed” at this level17See for example:
– Use of single particle ICP-MS to estimate silver nanoparticle penetration through baby porcine mucosa, Zanoni I et al, Nanotoxicology, 15(8): 1005-1015, 2021
– Nano-TiO2 penetration of oral mucosa: in vitro analysis using 3D organotypic human buccal mucosa models, Konstantinova V et al, Journal of Oral Pathology & Medicine, 46(3): 214-222, March 2017
– The buccal mucosa as a route for TiO2 nanoparticle uptake, Teubl BJ et al, Nanotoxicology, 9: 253-261, 2015
– Interactions between nano-TiO2 and the oral cavity: Impact of nanomaterial surface hydrophilicity/hydrophobicity, Teubl BJ et al, Journal of Hazardous Materials, 286: 298-305, 2015
– In vitro permeability of silver nanoparticles through porcine oromucosal membrane, Mauro M et al, Colloids Surf B Biointerfaces, 1;132:10-6, 2015
– Evaluation of a physiological in vitro system to study the transport of nanoparticles through the buccal mucosa, Roblegg E et al, Nanotoxicology, 1-15, 2011.
What happens to nanomaterials in the human body?
The small size of nanomaterials also explains their propensity to diffuse in the body through the nervous, blood and lymphatic systems. Nanomaterials can indeed cross the different physiological barriers: nasal barriers18Inhaled nanoparticles can travel via the olfactory nerves to the olfactory lobes (in the brain): Oberdörster et al, Translocation of inhaled ultrafine particles to the brain, 2004; Oberdörster et al, Nanotoxicology: an emerging discipline evolving from studies of ultrafine particles, Environ Health Perspect, 113(7) :823-839, 2005, bronchial / alveolar19Cf:
– Biopersistence and translocation to extrapulmonary organs of titanium dioxide nanoparticles after subacute inhalation exposure to aerosol in adult and elderly rats, Gaté L et al, Toxicol. Lett, 4; 265: 61-69, January 2017
– “Internalization and translocation of silica oxide and titanium oxide nanoparticles in bronchial epithelial, pulmonary endothelial and muscle cells” by Mornet S et al. and “Study of the passage of the air-blood barrier of carbon nanotubes after pulmonary exposure” by Czarny et al, in Participant file prepared for the Restitution of the National Research Program on Environment and Health at Work (PNREST), October 2015
– Pathophysiological impacts of inhaled nanoparticles, Baeza-Squiban A, Biology Today, 208 (2), 151-158, September 2014 (paragraph “The respiratory system, target of inhaled nanoparticles”)
– Nanomaterials, INRS, ED6050, September 2012, See our section on the risks associated with nanomaterials in food, placental20See in particular these few studies on the passage of nanomaterials through the placental barrier (non-exhaustive list):
– Titanium dioxide nanoparticles: E171 crosses the placental barrier, INRAE, October 7, 2020 ; Basal Ti level in the human placenta and meconium and evidence of a materno-foetal transfer of food-grade TiO2 nanoparticles in an ex vivo placental perfusion model, A. Guillard et al, Particle and Fibre Toxicology, 17(51), 2020
– Female fertility data lacking for nanomaterials, European Observatory of Nanomaterials, April 6, 2020 and A critical review of studies on the reproductive and developmental toxicity of nanomaterials, ECHA / Danish National Research Centre for the Working Environment, April 2020
– Ambient black carbon particles reach the fetal side of human placenta, Bové H et al, Nature Communications, volume 10, 2019
– Nanoparticle-induced neuronal toxicity across placental barriers is mediated by autophagy and dependent on astrocytes, Hawkins S J et al, Nature Nanotechnology, 13: 427-433, 2018
– Maternal exposure to nanosized titanium dioxide suppresses embryonic development in mice, Hong F et al, Int J Nanomedicine, 12: 6197-6204, 2017, cited by the High Council for Public Health (HCSP) : “In pregnant mice exposed orally to TiO2 nanoparticles between 0 and 17 days of gestation at doses up to 100 mg/kg bw/day, the concentration of Ti increases in the mother’s serum, in the placenta and in the fetus. Abnormalities in weight and skeletal development are also found in the fetus. These results indicate that TiO2 nanoparticles can cross the placental barrier in mice with consequences for fetal development.“Maternal exposure to TiO2 NPs” in Bilan des connaissances relatives aux effets des nanoparticules de dioxyde de titane (TiO2) sur la santé humaine; caractérisation de l’exposition des populations et mesures de gestion, HCSP, April 2018 (made public in June 2018)
– The toxicity, transport and uptake of nanoparticles in the in vitro BeWo b30 placental cell barrier model used within NanoTEST, Carreira C S et al, Nanotoxicology, 9 Suppl 1: 66-78, May 2015
– “Biometrology of ultrafine particles: application in two studies” (including one on placental translocation), by Rinaldo M et al, in Participant’s file prepared for the Restitution of the National Environment Health and Work Research Program (PNREST), October 2015
– The toxicity, transport and uptake of nanoparticles in the in vitro BeWo b30 placental cell barrier model used within NanoTEST, Nanotoxicology, Sept. 2013
– Kinetics of silica nanoparticles in the human placenta, Nanotoxicology, July 2013
– Nanotoxicology: Nanoparticles versus the placenta, Nature Nanotechnology, May 2011
– Barrier capacity of human placenta for nanosized materials, Environ Health Perspect, 2010
– Transfer of Quantum Dots from Pregnant Mice to Pups Across the Placental Barrier, Small, 2010
– Effects of prenatal exposure to surface-coated nanosized titanium dioxide (UV-Titan). A study in miceParticle and Fibre Toxicology, 2010, blood-brain barrier21The passage of nanoparticles in the brain has been demonstrated for nanoparticles of various types (silver, titanium dioxide (TiO2), manganese dioxide, iron oxides, iridium, carbon, polystyrene, etc.):
– Can the brain’s gatekeeper fight a nano-attack? E/ Valsami-Jones, EUON, September 2022 > Nanomaterials shape and form influences their ability to cross the blood brain barrier, University of Birmingham, July 2021 (press release) ; Biotransformation modulates the penetration of metallic nanomaterials across an artificial blood-brain barrier model, Guo Z et al, PNAS, 118 (28), July 2021
– Brain Inflammation, Blood Brain Barrier dysfunction and Neuronal Synaptophysin Decrease after Inhalation Exposure to Titanium Dioxide Nano-aerosol in Aging Rats, Disdier C et al, Scientific reports vol 7,1 12196, 2017
– Do nanoparticles harm the brain, Bencsik A., Pour la science, n°448, February 2015
– Is the brain safe from the impact of nanomaterial exposure, Anna Bencsik, Biology Today, 208 (2): 159-165, 2014
– PRESS RELEASE: Titanium dioxide nanoparticles alter, in vitro, the blood-brain barrier, CEA, October 26, 2011; related academic publication: In vitro evidence of dysregulation of blood-brain barrier function after acute and repeated/long-term exposure to TiO(2) nanoparticles, Brun E, Carrière M, Mabondzo A., Biomaterials, 33(3):886-96, January 2012.
In humans, researchers have recently demonstrated that metallic nanoparticles (magnetite) from polluted air reach our brain, crossing the blood-brain barrier specific to this organ (based on analyses of brain samples from 37 deceased people of all ages, all living in polluted cities: Mexico City and Manchester)22Cf. Magnetite pollution nanoparticles in the human brain, Maher BA et al, PNAS, July 2016(the barrier normally protects the brain from pathogens and toxins circulating in the blood).
Some nanomaterials can damage23Researchers at Imperial College London have shown, for example, that medium-sized nanoparticles (25-35 nm) generally adhere to the surface and cause some distortion, while small gold nanoparticles (5-10 nm) significantly deform cell membranes, sometimes bending them inwards with several stacked nanoparticles, causing tubular distortion. Cf. Size determines how nanoparticles affect biological membranes, Dunning, H., Imperial College London, September 17, 2020 (press release) and Size dependency of gold nanoparticles interacting with model membranes, Contini, C et al, Nature Communications Chemistry, 130, 2020 and even cross cell membranes24See in particular:
– Size determines how nanoparticles affect biological membranes, Dunning, H., Imperial College London, September 17, 2020 (and Size dependency of gold nanoparticles interacting with model membranes, Contini, C et al., Nature Communications Chemistry, 130, 2020)
– Singh, S et al, Endocytosis, oxidative stress and IL-8 expression in human lung epithelial cells upon treatment with fine and ultrafine TiO2: Role of the specific surface area and of surface methylation of the particles, Toxicology and Applied Pharmacology, 222 (2), 141-151, 2007
– Geiser, M et al, Ultrafine Particles Cross Cellular Membranes by Nonphagocytic Mechanisms in Lungs and in Cultured Cells, Environ Health Perspect, 113 (11), 1555-1560, 2005
– Limbach, LK et al, Oxide Nanoparticle Uptake in Human Lung Fibroblasts: Effects of Particle Size, Agglomeration, and Diffusion at Low Concentrations, Environmental Science & Technology, 39 (23), 9370-9376, 2005..
Nanomaterials (especially metallic nanoparticles) can accumulate over time:
- in the lymph nodes (leading to their chronic swelling)25See in particular:
– Scientists find that nanoparticles from tattoos travel inside the body, ESRF, September 12, 2017 (Synchrotron-based ν-XRF mapping and μ-FTIR microscopy enable to look into the fate and effects of tattoo pigments in human skin, Schreiver I et al, Scientific reports, 2017)
– University of Bradford (UK) press release and Friends of the Earth Australia article: Nanoparticles in tattoos could cause cancer, 4 November 2013 - in certain organs26See for example:
– Remote effects and biodistribution of pulmonary instilled silver nanoparticles in mice, Ferdous Z et al, NanoImpact, 22, April 2021
– Kidneys could be ‘dump’ for inhaled cerium dioxide nanoparticles, Chemical Watch, 7 April 2020 and Organ burden of inhaled nanoceria in a 2-year low-dose exposure study: dump or depot?, Tentschert J et al, Nanotoxicology, 2020
– Radiotracers for biokinetic studies with titanium nanoparticles, JRC, June 13, 2017
– British researchers have warned of the risks associated with the transfer of nanoparticles contained in tattoos to the blood, lymphatic vessels and various organs. See the University of Bradford (UK) press release and Friends of the Earth Australia article: Nanoparticles in tattoos could cause cancer, November 4, 2013
– Another study further suggests accumulation in the liver, kidney, heart and brain (of mice): Extrapulmonary transport of MWCNT following inhalation exposure, Mercer et al, Particle and Fibre Toxicology, 10:38, August 2013
– Researchers have shown that carbon nanotubes inhaled by mice are found in the spleen and liver, where they seem to persist: “Translocation of carbon nanotubes in secondary organs after pulmonary exposure in mice” in Participant file, Chemical substances and nanoparticles, ANSES, 2013 and Carbon Nanotube Translocation to Distant Organs after Pulmonary Exposure: Insights from in Situ 14C-Radiolabeling and Tissue Radioimaging, ACS Nano, 8 (6), 5715-5724, 2014
– Nanotechnologies and nanoparticles in food and feed, Afssa (now ANSES), March 2009: the digestive tract, liver and spleen are the main target organs. After oral administration, nanoparticles can also be found in the stomach, kidneys, liver, lungs, testicles, brain and blood. (digestive tract, liverSee for example:
– Nanotechnologies and nanoparticles in food and feed, Afssa (now ANSES), March 2009: the digestive tract, liver and spleen are the main target organs. After oral administration, nanoparticles can also be found in the stomach, kidneys, liver, lungs, testicles, brain and blood.
– Researchers have shown that carbon nanotubes inhaled by mice are found in the spleen and liver, where they seem to persist: “Translocation of carbon nanotubes in secondary organs after pulmonary exposure in mice” in Participant file, Chemical substances and nanoparticles, ANSES, 2013 and Carbon Nanotube Translocation to Distant Organs after Pulmonary Exposure: Insights from in Situ 14C-Radiolabeling and Tissue Radioimaging, ACS Nano, 8 (6), 5715-5724, 2014
– Another study further suggests accumulation in the liver, kidney, heart and brain (of mice): Extrapulmonary transport of MWCNT following inhalation exposure, Mercer et al, Particle and Fibre Toxicology, 10:38, August 2013
– Detection of titanium particles in human liver and spleen and possible health implicationsHeringa MB et al, Particle and Fibre Toxicology, 15:15, 2018 : des nanoparticles of titanium dioxide (TiO2) have been detected in the liver and spleen of 15 humans (not only in laboratory rats). In half of the cases, the levels were above the level considered safe for the liver. (spleen27See for example:
– Nanotechnologies and nanoparticles in food and feed, Afssa (now ANSES), March 2009: the digestive tract, liver and spleen are the main target organs. After oral administration, nanoparticles can also be found in the stomach, kidneys, liver, lungs, testicles, brain and blood.
– Researchers have shown that carbon nanotubes inhaled by mice are found in the spleen and liver, where they seem to persist: “Translocation of carbon nanotubes in secondary organs after pulmonary exposure in mice” in Participant file, Chemical substances and nanoparticles, ANSES, 2013 and Carbon Nanotube Translocation to Distant Organs after Pulmonary Exposure: Insights from in Situ 14C-Radiolabeling and Tissue Radioimaging, ACS Nano, 8 (6), 5715-5724, 2014, stomach, kidneys, lungs, testicles, heart, brain28See for example:
– In the brain, nanoparticles can enter cells of all types: they have been observed in neurons, astrocytes (which perform various important functions, including providing nutrients to neurons) and microglial cells (immune cells that protect the nervous system from pathogens): see Bencsik A, Is the brain safe from the impact of nanomaterial exposure, Biology Today, 208 (2), 159-165, September 2014
– Nanotechnologies and nanoparticles in food and feed, Afssa (now ANSES), March 2009: the digestive tract, liver and spleen are the main target organs. After oral administration, nanoparticles can also be found in the stomach, kidneys, liver, lungs, testicles, brain and blood.
– Another study further suggests accumulation in the liver, kidney, heart and brain (of mice): Extrapulmonary transport of MWCNT following inhalation exposure, Mercer et al, Particle and Fibre Toxicology, 10:38, 2013) - and even inside the cells29See for example:
– Rashid MM et al, Influence of Titanium Dioxide Nanoparticles on Human Health and the Environment, Nanomaterials, 11(9):2354, 2021: TiO2 nanoparticles, up to 25 nm in size, have been found in different types of human cells: keratinocytes, lung cells, lymphocytes, macrophages and hepatocytes
– Hussain Baeza-Squiban A, Pathophysiological impacts of inhaled nanoparticles, Biology Today, 208 (2), 151-158, September 2014 : “nanoparticles are most often present in vesicles but sometimes free in the cytoplasm without it always being known whether this is due to a release of nanoparticles from vesicles whose membrane has broken or to an entry by diffusion of nanoparticles. Depending on the cell types considered and the nanoparticles studied, the internalization pathways of nanoparticles can be very varied, ranging from passive diffusion to active mechanisms such as phagocytosis, macropinocytosis or clathrin- or caveolin-dependent endocytosis.”
– Bencsik A, Is the brain safe from the impact of exposure to nanomaterials?, Biology Today, 208 (2), 159-165, September 2014: in the brain, nanoparticles can enter cells of all types; for example, they have been found in the cytoplasm and nucleus of glial and neuronal cells.
– Translocation mechanisms of chemically functionalised carbon nanotubes across plasma membranes, Biomaterials, 33(11): 3334-43, April 2012.
Some useful information
- The ability to cross physiological barriers, the affinity of nanomaterials for a given type of organ or cell, as well as their toxicity, vary greatly from one nanomaterial to another because they are highly dependent on their physicochemical characteristics.
- A scientific literature review, published in June 2023, provides the latest information on this issue : Cf. Determinants and mechanisms of inorganic nanoparticle translocation across mammalian biological barriers, Cary C & Stapleton P, Archives of Toxicology, 97, 2023.
- Some nanomaterials can be degraded and/or eliminated through urine and feces, but that does not mean that they are not a problem. When the body has to get rid of chemicals, it implements a number of detoxification strategies whose processes themselves can lead to toxicity30A study on Oryzias latipes (fish) livers showed detoxification mechanisms (induction of metallothionein, CYP 450, GST, etc.) Cf. Evaluation of the toxic impact of silver nanoparticles on Japanese medaka (Oryzias latipes), Chae et al, Aquat Toxicol, 94(4):320-7, 2009.
- Many very important questions are still unresolved today. How to evaluate the elimination or degradation processes of nanoparticles? How to identify degradation products and their effects? How to control the persistence of nanoparticles in organs? What is the link between biopersistence, reactivity, degradation and toxicity of nanoparticles? Despite advances in nanometrology31See for example: An analytical workflow for dynamic characterization and quantification of metal-bearing nanomaterials in biological matrices, Monikh FA et al, Nature protocols, 2022, there are currently no clear answers. The degradation/elimination rate of nanoparticles in vivo (a few months) is much slower than that observed in vitro (a few hours or days)32Kolosnjaj-Tabi J et al, Life cycle of magnetic nanoparticles in the body, Biology Today, 208 (2), 177-190, September 2014.
How long can nanomaterials persist in the body? It is not known today and the answer will be different again depending on the nanomaterial considered.
Finally, what are the effects on human health once the nanomaterials or their residues have penetrated our organs and cells? There are still many uncertainties, but the initial results are quite worrying.
Any questions or comments? This information sheet compiled by AVICENN is intended to be completed and updated. Please feel free to contribute.
Upcoming Nano Agenda

- Advanced Characterization Techniques in Nanomaterials and Nanotechnology
- 10th European Congress on Advanced Nanotechnology and Nanomaterials
- Website: https://nanomaterialsconference.com
- Training intended for occupational physicians, occupational risk prevention specialists (IPRP), company prevention specialists, prevention department staff from Carsat, Cramif and CGSS, institutional prevention specialists (Dreets, Dreal, MSA…).
- Organizer: French National institute of research and security (INRS)
- October 6 to 10, 2025
- Website: www.inrs.fr/…/formation/…JA1030_2025
File originally created in November 2013
Notes and references
- 1See for example:
– Human study reveals nanoparticles cross from lungs into blood – Gold nanoparticles accumulate in arterial plaques, Chemical Watch, May 2017 (see Inhaled Nanoparticles Accumulate at Sites of Vascular Disease, Miller MR et al, ACS Nano, 11(5): 4542-4552, April 2017)
– Uranium nanoparticles cross the pulmonary barrier, Aktis (IRSN), October-December 2013
– Les nanomatériaux, INRS, ED6050, September 2012. - 2See Potential exposure of adults and children to particles from resuspended nano-enabled consumer sprays, He R at al., Science of The Total Environment, 924, May 2024
- 3See Les nanomatériaux, INRS, ED6050, September 2012
- 4Cf. Oral exposure to titanium dioxide (TiO2) nanoparticles: from crossing the oral and intestinal epithelium to fate and effects in the body, Bettini S and Houdeau E, Biology Today, September 2014
- 5See in particular:
For the money:
– Yves-Jacques Schneider in Nanoparticles dans nos assiettes: le Grand Secret, RTS, 3 May 2016 (intervention 19′)
– Walczak AP et al, Behaviour of silver nanoparticles and silver ions in an in vitro human gastrointestinal digestion model, Nanotoxicology, 7(7), 2012
For silica:
– Eric Houdeau and Marie-Hélène Ropers (INRAE), Nanomaterials in food. What functions and applications? What are the risks?, NanoResp, October 2015
– Peters R & Bouwmeester H, Fate of nano-sized silica during in vitro digestion, RIKILT, 2013 - 6See for example Nanotherapeutic approaches for transdermal drug delivery systems and their biomedical applications, Khan SU et al., European Polymer Journal, 207, 2024
- 7See for example “Our cosmetics are worth their weight in gold,” Avantages Magazine, December 7, 2018: “By passing through the skin barrier, gold would fight against oxidative stress due to pollution and UV, thus limiting wrinkles. It would also be able to fight against spots and boost the skin’s immune system.”
- 8Cf. for example:
– Ex vivo skin diffusion and decontamination studies of titanium dioxide nanoparticles, Tarantini A et al., Toxicology in Vitro, 101, 105918, 2024
– A critical review investigating the use of nanoparticles in cosmetic skin products, Piluk T et al., Environ. Sci.: Nano, 11, 3674-3692, 2024
– SCCS Guidance on the safety assessment of nanomaterials in cosmetics, Scientific Committee on Consumer Safety (SCCS), 2nd revision, June 2023
– Toxicity assessment of nanoparticles in contact with the skin, Zaiter T et al., Journal of Nanoparticle Research, 24 (149), 2022
– A critical review of the factors determining dermal absorption of nanomaterials and available tools for the assessment of dermal absorption, Triskelion & RIVM for EUON, 2020
– Toxicity evaluation of TiO2 nanoparticles on the 3D skin model: a systematic review, Sanches PL et al., Front Bioeng Biotechnol, 8, 2020
– NanoTiO2 Sunscreen Does Not Prevent Systemic Oxidative Stress Caused by UV Radiation and a Minor Amount of NanoTiO2 is Absorbed in Humans, Pelclova D et al., Nanomaterials, 9(6), 888, 2019
– NanoTiO2 Sunscreen Does Not Prevent Systemic Oxidative Stress Caused by UV Radiation and a Minor Amount of NanoTiO2 is Absorbed in Humans, Pelclova D et al, Nanomaterials, 9(6), 888, 2019
– Support for the Safe Use of Zinc Oxide Nanoparticle Sunscreens: Lack of Skin Penetration or Cellular Toxicity after Repeated Application in Volunteers, Mohammed YH, Journal of Investigative Dermatology, 139(2): 308-315, February 2019
– Sunscreen, nanoparticles and frontal alopecia, Synchrotron sun, press release, February 2018 (see also academic article in English: Postmenopausal fibrosing frontal alopecia: a lichenoid reaction to titanium dioxide nanoparticles in hair follicles?, Gary C et al., Annals of Dermatology and Venereology, 144 (12), S206, 2017)
– Skin exposure to nanoparticles and possible sensitization risk, Larese Filon in Allergy and Immunotoxicology in Occupational Health, Otsuki, T, Petrarca, C, Di Gioacchino, M (eds) 143-152, 2017
– Titanium dioxide and silver nanoparticles- Dermal exposure, Proust N, Techniques de l’Ingénieur, January 2017
– Bioengineered sunscreen blocks skin penetration and toxicity, NIBIB, December 2015
– Is the skin really impervious to nanoparticles, Vinches L and Halle S, Bulletin de veille scientifique, No. 27, ANSES, September 2015
– A review of critical factors for assessing the dermal absorption of metal oxide nanoparticles from sunscreens applied to humans and a research strategy to address current deficiencies, Gulson, B, Arch. Toxicol. 89, 1909–1930, 2015
– Nanoparticles skin absorption: New aspects for a safety profile evaluation, Larese Filon F et al. Regulatory Toxicology and Pharmacology, 2015: according to this literature review:
. nanoparticles smaller than 4 nm in diameter can penetrate and impregnate intact skin,
. nanoparticles with a diameter between 4 and 20 nm can potentially penetrate intact and injured skin,
. nanoparticles with a diameter between 21 and 45 nm can only penetrate and impregnate injured skin,
. nanoparticles with a diameter greater than 45 nm cannot penetrate or impregnate the skin.
– Other aspects play an important role, especially for metallic nanoparticles, namely their dissolution in physiological media, which can cause local and systemic effects, their sensitizing or toxic potential and the tendency to create aggregates.
– Interactions of Skin with Gold Nanoparticles of Different Surface Charge, Shape, and Functionality, Fernandes R et al, Small, October 2014
– Dermal exposure potential from textiles that contain silver nanoparticles, International Journal of Occupational and Environmental Health, 20(3), July 2013
– Dermal Absorption of Nanomaterials, Danish Environmental Protection Agency, 2013
– Occupational exposure to nanoparticles and skin protection, Archives of Occupational and Environmental Diseases, 74(5), 488-498, November 2013
– Reigniting the controversy over the ability of nanoparticles to cross the skin barrier, veillenanos.co.uk, 3 October 2012
– Titanium dioxide and zinc oxide nanoparticles in cosmetic products: State of knowledge on skin penetration, genotoxicity and carcinogenesis – Information point AFSSAPS, June 14, 2011 (see pp. 28-29 of the state of knowledge report for a presentation of the Gulson study): the French Agency for the Sanitary Safety of Health Products (Afssaps) noted that scientific studies did not show significant cutaneous penetration of titanium dioxide (TiO2) nanoparticles for healthy skin, but did not allow for any conclusion in either direction for damaged skin. Afssaps has therefore recommended not to apply creams containing nano TiO2 on injured skin (for example, sunburn) because of the potential risks to human health; it has also advised against using cosmetics containing nanoparticles in spray form on the face or in closed premises - 9A synthesis was published in May 2020: Are nanomaterials getting under your skin? RIVM & RPA consortium of Triskelion, ECHA, EUON, May 2020: experts from the Netherlands commissioned by the European Chemicals Agency (ECHA) to analyze research on dermal absorption of nanomaterials highlight the lack of comparable and quality data and recommend well-organized and structured research programs in line with the OECD testing guidelines.
A study, also published in May 2020, reports interesting results: Penetration of Zinc into Human Skin after Topical Application of Nano Zinc Oxide Used in Commercial Sunscreen Formulations, Holmes AM et a., ACS Appl. Bio Mater., 2020 - 10Research work is underway in 2021 at the CEA in Grenoble in particular, on the development of an experimental model for the study of skin decontamination after exposure to metal nanoparticles (NaPeauLi), funded under the 2019 Anses APR-EST.
- 11Cf. Sunscreen, nanoparticles, and frontal alopecia, Synchrotron sun, February 2018
- 12See our inventories of marketed products containing nanomaterials, or for example: Testing for HIV with the naked eye or countering it with a cream? January 2014 and Use of silver nanoparticles increased inhibition of cell-associated HIV-1 infection by neutralizing antibodies developed against HIV-1 envelope proteins, Journal of Nanobiotechnology, 9:38, 2011
- 13See in particular:
– Tattoo : nanoparticles, I have you in my skin, Science & Vie, 20 October 2021
– Tattoo inks and permanent make-up, ECHA, 2020 ?
– After the ink… Tattoos: needles could cause allergies, Allô Docteurs, 27 August 2019 and Metal particles abraded from tattooing needles travel inside the body, Synchrotron de Grenoble, 26 August 2019
– Scientists find that nanoparticles from tattoos travel inside the body, ESRF, September 12, 2017 (Synchrotron-based ν-XRF mapping and μ-FTIR microscopy enable to look into the fate and effects of tattoo pigments in human skin, Schreiver I et al, Scientific reports, 2017); professionals have responded by downplaying the risks, while still denouncing “the vagueness maintained by the industrialists supplying the pigments (CI = Color index) to the ink manufacturers, under cover of commercial secrecy. Our national distributors, respectful of the requirements imposed by the law, do not have themselves details on the CI of the labels. It is indeed today the only zone of shade on our tattoo inks. The real evolution expected from now on by the tattooers and the tattooed aims at a bigger transparency of the pigments suppliers… For a better knowledge of tattoo products”. Nanoparticles : Don’t panic, Tattoo Magazine, n°119, November-December 2017
– Size and metal composition characterization of nano- and microparticles in tattoo inks by a combination of analytical techniques, Bocca B et al, J. Anal. At. Spectrom.,32, 616-628, January 2017
– University of Bradford (UK) press release and Friends of the Earth Australia article: Nanoparticles in tattoos could cause cancer, 4 November 2013 - 14See in particular:
–New Quality-Control Investigations on Vaccines: Microand Nanocontamination, Gatti AM and Montanari S, International Journal of Vaccines and Vaccination, 4(1), 2017
-Comparative study of particle and element testing in vaccines and other injectable health products, ANSM, May 2016 - 15“Are vaccines “contaminated by toxic nanoparticles”?, Le Monde, July 19, 2017
- 16See for example:
– Vaccine leads under study against covid-19, some examples of which are compiled on our Nano and covid-19 page
– Nanotechnologies to produce vaccines faster, Le Soir, November 8, 2021
– Vaxinano, a biotechnology company established in 2016, specializes in the preclinical and pharmaceutical development of prophylactic and therapeutic vaccines for infectious diseases for the human and animal health markets.
– Glycovax Pharma files patent application for new semi-synthetic breast cancer vaccine, Glycovax Pharma, April 4, 2018
– Vaxinano develops first toxoplasmosis vaccine, Les Echos, Sept. 5, 2017
– A method for vaccines to no longer need cold storage, Sciences & Avenir, December 2016
– Applications of nanotechnology to medicine, LEEM, February 13, 2014
– Vaccines based on nanoparticles, Les Echos, October 3, 2007, mainly at the research and development stage, and some are already being marketed. - 17See for example:
– Use of single particle ICP-MS to estimate silver nanoparticle penetration through baby porcine mucosa, Zanoni I et al, Nanotoxicology, 15(8): 1005-1015, 2021
– Nano-TiO2 penetration of oral mucosa: in vitro analysis using 3D organotypic human buccal mucosa models, Konstantinova V et al, Journal of Oral Pathology & Medicine, 46(3): 214-222, March 2017
– The buccal mucosa as a route for TiO2 nanoparticle uptake, Teubl BJ et al, Nanotoxicology, 9: 253-261, 2015
– Interactions between nano-TiO2 and the oral cavity: Impact of nanomaterial surface hydrophilicity/hydrophobicity, Teubl BJ et al, Journal of Hazardous Materials, 286: 298-305, 2015
– In vitro permeability of silver nanoparticles through porcine oromucosal membrane, Mauro M et al, Colloids Surf B Biointerfaces, 1;132:10-6, 2015
– Evaluation of a physiological in vitro system to study the transport of nanoparticles through the buccal mucosa, Roblegg E et al, Nanotoxicology, 1-15, 2011 - 18Inhaled nanoparticles can travel via the olfactory nerves to the olfactory lobes (in the brain): Oberdörster et al, Translocation of inhaled ultrafine particles to the brain, 2004; Oberdörster et al, Nanotoxicology: an emerging discipline evolving from studies of ultrafine particles, Environ Health Perspect, 113(7) :823-839, 2005
- 19Cf:
– Biopersistence and translocation to extrapulmonary organs of titanium dioxide nanoparticles after subacute inhalation exposure to aerosol in adult and elderly rats, Gaté L et al, Toxicol. Lett, 4; 265: 61-69, January 2017
– “Internalization and translocation of silica oxide and titanium oxide nanoparticles in bronchial epithelial, pulmonary endothelial and muscle cells” by Mornet S et al. and “Study of the passage of the air-blood barrier of carbon nanotubes after pulmonary exposure” by Czarny et al, in Participant file prepared for the Restitution of the National Research Program on Environment and Health at Work (PNREST), October 2015
– Pathophysiological impacts of inhaled nanoparticles, Baeza-Squiban A, Biology Today, 208 (2), 151-158, September 2014 (paragraph “The respiratory system, target of inhaled nanoparticles”)
– Nanomaterials, INRS, ED6050, September 2012, See our section on the risks associated with nanomaterials in food - 20See in particular these few studies on the passage of nanomaterials through the placental barrier (non-exhaustive list):
– Titanium dioxide nanoparticles: E171 crosses the placental barrier, INRAE, October 7, 2020 ; Basal Ti level in the human placenta and meconium and evidence of a materno-foetal transfer of food-grade TiO2 nanoparticles in an ex vivo placental perfusion model, A. Guillard et al, Particle and Fibre Toxicology, 17(51), 2020
– Female fertility data lacking for nanomaterials, European Observatory of Nanomaterials, April 6, 2020 and A critical review of studies on the reproductive and developmental toxicity of nanomaterials, ECHA / Danish National Research Centre for the Working Environment, April 2020
– Ambient black carbon particles reach the fetal side of human placenta, Bové H et al, Nature Communications, volume 10, 2019
– Nanoparticle-induced neuronal toxicity across placental barriers is mediated by autophagy and dependent on astrocytes, Hawkins S J et al, Nature Nanotechnology, 13: 427-433, 2018
– Maternal exposure to nanosized titanium dioxide suppresses embryonic development in mice, Hong F et al, Int J Nanomedicine, 12: 6197-6204, 2017, cited by the High Council for Public Health (HCSP) : “In pregnant mice exposed orally to TiO2 nanoparticles between 0 and 17 days of gestation at doses up to 100 mg/kg bw/day, the concentration of Ti increases in the mother’s serum, in the placenta and in the fetus. Abnormalities in weight and skeletal development are also found in the fetus. These results indicate that TiO2 nanoparticles can cross the placental barrier in mice with consequences for fetal development.“Maternal exposure to TiO2 NPs” in Bilan des connaissances relatives aux effets des nanoparticules de dioxyde de titane (TiO2) sur la santé humaine; caractérisation de l’exposition des populations et mesures de gestion, HCSP, April 2018 (made public in June 2018)
– The toxicity, transport and uptake of nanoparticles in the in vitro BeWo b30 placental cell barrier model used within NanoTEST, Carreira C S et al, Nanotoxicology, 9 Suppl 1: 66-78, May 2015
– “Biometrology of ultrafine particles: application in two studies” (including one on placental translocation), by Rinaldo M et al, in Participant’s file prepared for the Restitution of the National Environment Health and Work Research Program (PNREST), October 2015
– The toxicity, transport and uptake of nanoparticles in the in vitro BeWo b30 placental cell barrier model used within NanoTEST, Nanotoxicology, Sept. 2013
– Kinetics of silica nanoparticles in the human placenta, Nanotoxicology, July 2013
– Nanotoxicology: Nanoparticles versus the placenta, Nature Nanotechnology, May 2011
– Barrier capacity of human placenta for nanosized materials, Environ Health Perspect, 2010
– Transfer of Quantum Dots from Pregnant Mice to Pups Across the Placental Barrier, Small, 2010
– Effects of prenatal exposure to surface-coated nanosized titanium dioxide (UV-Titan). A study in miceParticle and Fibre Toxicology, 2010 - 21The passage of nanoparticles in the brain has been demonstrated for nanoparticles of various types (silver, titanium dioxide (TiO2), manganese dioxide, iron oxides, iridium, carbon, polystyrene, etc.):
– Can the brain’s gatekeeper fight a nano-attack? E/ Valsami-Jones, EUON, September 2022 > Nanomaterials shape and form influences their ability to cross the blood brain barrier, University of Birmingham, July 2021 (press release) ; Biotransformation modulates the penetration of metallic nanomaterials across an artificial blood-brain barrier model, Guo Z et al, PNAS, 118 (28), July 2021
– Brain Inflammation, Blood Brain Barrier dysfunction and Neuronal Synaptophysin Decrease after Inhalation Exposure to Titanium Dioxide Nano-aerosol in Aging Rats, Disdier C et al, Scientific reports vol 7,1 12196, 2017
– Do nanoparticles harm the brain, Bencsik A., Pour la science, n°448, February 2015
– Is the brain safe from the impact of nanomaterial exposure, Anna Bencsik, Biology Today, 208 (2): 159-165, 2014
– PRESS RELEASE: Titanium dioxide nanoparticles alter, in vitro, the blood-brain barrier, CEA, October 26, 2011; related academic publication: In vitro evidence of dysregulation of blood-brain barrier function after acute and repeated/long-term exposure to TiO(2) nanoparticles, Brun E, Carrière M, Mabondzo A., Biomaterials, 33(3):886-96, January 2012 - 22Cf. Magnetite pollution nanoparticles in the human brain, Maher BA et al, PNAS, July 2016
- 23Researchers at Imperial College London have shown, for example, that medium-sized nanoparticles (25-35 nm) generally adhere to the surface and cause some distortion, while small gold nanoparticles (5-10 nm) significantly deform cell membranes, sometimes bending them inwards with several stacked nanoparticles, causing tubular distortion. Cf. Size determines how nanoparticles affect biological membranes, Dunning, H., Imperial College London, September 17, 2020 (press release) and Size dependency of gold nanoparticles interacting with model membranes, Contini, C et al, Nature Communications Chemistry, 130, 2020
- 24See in particular:
– Size determines how nanoparticles affect biological membranes, Dunning, H., Imperial College London, September 17, 2020 (and Size dependency of gold nanoparticles interacting with model membranes, Contini, C et al., Nature Communications Chemistry, 130, 2020)
– Singh, S et al, Endocytosis, oxidative stress and IL-8 expression in human lung epithelial cells upon treatment with fine and ultrafine TiO2: Role of the specific surface area and of surface methylation of the particles, Toxicology and Applied Pharmacology, 222 (2), 141-151, 2007
– Geiser, M et al, Ultrafine Particles Cross Cellular Membranes by Nonphagocytic Mechanisms in Lungs and in Cultured Cells, Environ Health Perspect, 113 (11), 1555-1560, 2005
– Limbach, LK et al, Oxide Nanoparticle Uptake in Human Lung Fibroblasts: Effects of Particle Size, Agglomeration, and Diffusion at Low Concentrations, Environmental Science & Technology, 39 (23), 9370-9376, 2005. - 25See in particular:
– Scientists find that nanoparticles from tattoos travel inside the body, ESRF, September 12, 2017 (Synchrotron-based ν-XRF mapping and μ-FTIR microscopy enable to look into the fate and effects of tattoo pigments in human skin, Schreiver I et al, Scientific reports, 2017)
– University of Bradford (UK) press release and Friends of the Earth Australia article: Nanoparticles in tattoos could cause cancer, 4 November 2013 - 26See for example:
– Remote effects and biodistribution of pulmonary instilled silver nanoparticles in mice, Ferdous Z et al, NanoImpact, 22, April 2021
– Kidneys could be ‘dump’ for inhaled cerium dioxide nanoparticles, Chemical Watch, 7 April 2020 and Organ burden of inhaled nanoceria in a 2-year low-dose exposure study: dump or depot?, Tentschert J et al, Nanotoxicology, 2020
– Radiotracers for biokinetic studies with titanium nanoparticles, JRC, June 13, 2017
– British researchers have warned of the risks associated with the transfer of nanoparticles contained in tattoos to the blood, lymphatic vessels and various organs. See the University of Bradford (UK) press release and Friends of the Earth Australia article: Nanoparticles in tattoos could cause cancer, November 4, 2013
– Another study further suggests accumulation in the liver, kidney, heart and brain (of mice): Extrapulmonary transport of MWCNT following inhalation exposure, Mercer et al, Particle and Fibre Toxicology, 10:38, August 2013
– Researchers have shown that carbon nanotubes inhaled by mice are found in the spleen and liver, where they seem to persist: “Translocation of carbon nanotubes in secondary organs after pulmonary exposure in mice” in Participant file, Chemical substances and nanoparticles, ANSES, 2013 and Carbon Nanotube Translocation to Distant Organs after Pulmonary Exposure: Insights from in Situ 14C-Radiolabeling and Tissue Radioimaging, ACS Nano, 8 (6), 5715-5724, 2014
– Nanotechnologies and nanoparticles in food and feed, Afssa (now ANSES), March 2009: the digestive tract, liver and spleen are the main target organs. After oral administration, nanoparticles can also be found in the stomach, kidneys, liver, lungs, testicles, brain and blood. (digestive tract, liverSee for example:
– Nanotechnologies and nanoparticles in food and feed, Afssa (now ANSES), March 2009: the digestive tract, liver and spleen are the main target organs. After oral administration, nanoparticles can also be found in the stomach, kidneys, liver, lungs, testicles, brain and blood.
– Researchers have shown that carbon nanotubes inhaled by mice are found in the spleen and liver, where they seem to persist: “Translocation of carbon nanotubes in secondary organs after pulmonary exposure in mice” in Participant file, Chemical substances and nanoparticles, ANSES, 2013 and Carbon Nanotube Translocation to Distant Organs after Pulmonary Exposure: Insights from in Situ 14C-Radiolabeling and Tissue Radioimaging, ACS Nano, 8 (6), 5715-5724, 2014
– Another study further suggests accumulation in the liver, kidney, heart and brain (of mice): Extrapulmonary transport of MWCNT following inhalation exposure, Mercer et al, Particle and Fibre Toxicology, 10:38, August 2013
– Detection of titanium particles in human liver and spleen and possible health implicationsHeringa MB et al, Particle and Fibre Toxicology, 15:15, 2018 : des nanoparticles of titanium dioxide (TiO2) have been detected in the liver and spleen of 15 humans (not only in laboratory rats). In half of the cases, the levels were above the level considered safe for the liver. - 27See for example:
– Nanotechnologies and nanoparticles in food and feed, Afssa (now ANSES), March 2009: the digestive tract, liver and spleen are the main target organs. After oral administration, nanoparticles can also be found in the stomach, kidneys, liver, lungs, testicles, brain and blood.
– Researchers have shown that carbon nanotubes inhaled by mice are found in the spleen and liver, where they seem to persist: “Translocation of carbon nanotubes in secondary organs after pulmonary exposure in mice” in Participant file, Chemical substances and nanoparticles, ANSES, 2013 and Carbon Nanotube Translocation to Distant Organs after Pulmonary Exposure: Insights from in Situ 14C-Radiolabeling and Tissue Radioimaging, ACS Nano, 8 (6), 5715-5724, 2014 - 28See for example:
– In the brain, nanoparticles can enter cells of all types: they have been observed in neurons, astrocytes (which perform various important functions, including providing nutrients to neurons) and microglial cells (immune cells that protect the nervous system from pathogens): see Bencsik A, Is the brain safe from the impact of nanomaterial exposure, Biology Today, 208 (2), 159-165, September 2014
– Nanotechnologies and nanoparticles in food and feed, Afssa (now ANSES), March 2009: the digestive tract, liver and spleen are the main target organs. After oral administration, nanoparticles can also be found in the stomach, kidneys, liver, lungs, testicles, brain and blood.
– Another study further suggests accumulation in the liver, kidney, heart and brain (of mice): Extrapulmonary transport of MWCNT following inhalation exposure, Mercer et al, Particle and Fibre Toxicology, 10:38, 2013 - 29See for example:
– Rashid MM et al, Influence of Titanium Dioxide Nanoparticles on Human Health and the Environment, Nanomaterials, 11(9):2354, 2021: TiO2 nanoparticles, up to 25 nm in size, have been found in different types of human cells: keratinocytes, lung cells, lymphocytes, macrophages and hepatocytes
– Hussain Baeza-Squiban A, Pathophysiological impacts of inhaled nanoparticles, Biology Today, 208 (2), 151-158, September 2014 : “nanoparticles are most often present in vesicles but sometimes free in the cytoplasm without it always being known whether this is due to a release of nanoparticles from vesicles whose membrane has broken or to an entry by diffusion of nanoparticles. Depending on the cell types considered and the nanoparticles studied, the internalization pathways of nanoparticles can be very varied, ranging from passive diffusion to active mechanisms such as phagocytosis, macropinocytosis or clathrin- or caveolin-dependent endocytosis.”
– Bencsik A, Is the brain safe from the impact of exposure to nanomaterials?, Biology Today, 208 (2), 159-165, September 2014: in the brain, nanoparticles can enter cells of all types; for example, they have been found in the cytoplasm and nucleus of glial and neuronal cells.
– Translocation mechanisms of chemically functionalised carbon nanotubes across plasma membranes, Biomaterials, 33(11): 3334-43, April 2012 - 30A study on Oryzias latipes (fish) livers showed detoxification mechanisms (induction of metallothionein, CYP 450, GST, etc.) Cf. Evaluation of the toxic impact of silver nanoparticles on Japanese medaka (Oryzias latipes), Chae et al, Aquat Toxicol, 94(4):320-7, 2009
- 31See for example: An analytical workflow for dynamic characterization and quantification of metal-bearing nanomaterials in biological matrices, Monikh FA et al, Nature protocols, 2022
- 32Kolosnjaj-Tabi J et al, Life cycle of magnetic nanoparticles in the body, Biology Today, 208 (2), 177-190, September 2014